Plant Proteins
Plant proteins (also known as vegetable proteins) and their products have been consumed for centuries as the main protein source in Asia and Middle Eastern countries. With the growing interest in reducing the consumption of animal products for health, environmental and economic reasons, plant proteins are making up a higher proportion of the human diet in recent years. The chart below shows some of the plants that contain protein in sufficient quantity and quality that are attracting attention. 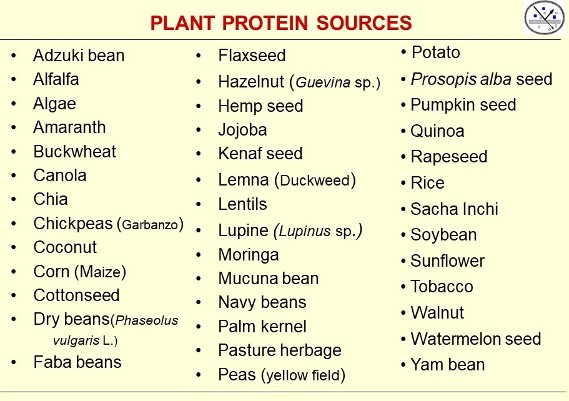
The challenges with using plant proteins in formulated food products include (a) the relatively low protein content of many protein sources : many legumes, leaves and oilseeds are 9 - 25% protein (N x 6.25) on a dry basis, although some can be as high as 42% dry basis, and (b) some may contain undesirable components that should be removed or reduced to increase the usefulness and functionality of the protein (almost all sources of plant protein share this problem). For example, some contain oligosaccharides that have been implicated with gastrointestinal distress. Lipid-lipoxygenase interactions can give rise to painty and/or oxidized off-flavors. Phytic acid forms insoluble chelates with minerals and can form complexes with proteins that reduce bioavailability of the minerals and proteins. Trypsin inhibitors are proteinaceous compounds that affect the efficiency of protein digestion.
Protein concentrates and isolates
Many of the above limitations can be avoided by selectively extracting the protein from the raw material, followed by purification and concentration of the protein. Traditional techniques involve alkali extraction, heat treatment, precipitation by the addition of acid or alcohol, and centrifugation or filtration to separate the protein from the other components. Most take advantage of the differences in solubility of the protein at various pH values as shown in the plot below. The first task is to solubilize the protein to allow for easier separation from non-protein components such as fiber and starch. The native pH of most plant proteins (e.g., when ground material is suspended in clean water) is 6.4 – 6.8. The plot suggests that either low pH or high pH can increase the solubility of the protein and thus increase yield. High pH solubilization is preferred using sodium hydroxide (NaOH) as the alkali. After separation of the soluble proteins, an acid such as hydrochloric, sulfuric or citric is added to precipitate the protein at its isoelectric pH.
Protein “concentrates” are generally defined as containing at least 65% protein on a dry basis(d.b.) where protein is expressed as nitrogen content (N) x 6.25. “Isolates” contain typically 85% or higher protein d.b. 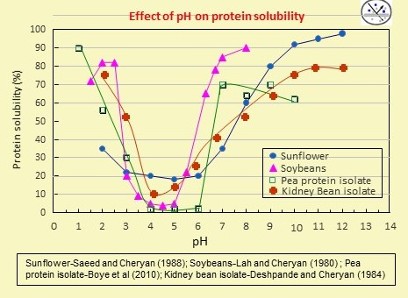
A typical process for producing protein concentrates and isolates is shown below. This process is from raw materials such as seeds, beans, nuts, pulses, lentils, peas, etc. A modified process is used for leafy materials (e.g., alfalfa, moringa, duckweed, switchgrass/pasture herbage) that require different upstream unit operations. Oilseeds (e.g., soybeans, sunflower, corn) with relatively high oil content require a defatting/oil extraction step ahead of protein extraction. On the other hand, yellow field peas, dry beans and the like with fat contents less than 2% d.b. generally have no defatting or oil extraction. However, not removing oil will result in high fat (4 - 8% d.b.) in the protein concentrate and isolate compared to starting with defatted meal.
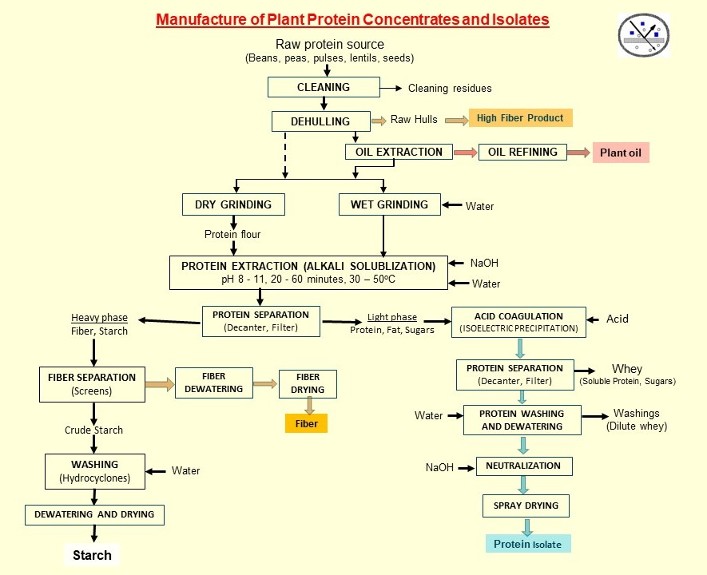
Regardless of the protein source, the main objective is to remove non-protein components in a stepwise manner:
(1) Cleaning: This removes non-plant material and any foreign matter that is not specifically the raw material of interest.
(2) Dehulling: This is to remove the outer tough shell that is mostly fiber. The hulls are frequently removed by dry methods although wet methods can also be used.
(3) Oil extraction: Physical methods such as pressing can be used but will result in much higher oil in the meal. Chemical methods with organic solvents such as hexane or ethanol will result in higher oil yields and much lower residual oil in the meal.
(4) Grinding/size reduction: Dry-ground dehulled flour or meal are most commonly used for plant protein concentrates and isolates. Regardless of wet- or dry-grinding, particle size of the flour/meal affects the efficiency of protein extraction and should be optimized.
(5) Protein extraction: The ground flour or meal is added to water, usually in a ratio of 1:5 – 1:20 of flour: water. The greater the ratio, the higher the yield of protein, but it also means a greater amount of liquid stream to handle in subsequent unit operations and more water to remove by dewatering or drying at the end of the process. Extraction is usually done in stirred tanks as a batch process. Other parameters that have to be optimized include pH, time and temperature of extraction.
(6) Protein separation; The slurry is subjected to either filtration or centrifugation to separate the soluble components (protein, soluble salts, sugars, minor components, and oil) from the insoluble components (fiber, starch, insoluble salts). Centrifuges (decanters) will result in two streams: the centrate or light phase containing the soluble components (protein, oil, sugars, soluble salts) and a heavy phase (sometimes termed the “sludge”, “curd”, “residue” or “solids”) containing the denser insoluble components (fiber, starch). Similarly, a filter or membrane with the appropriate screen/mesh or pore size can be used which will result in a filtrate or permeate stream containing the protein and soluble permeable components, and a filter cake or retentate stream containing the larger particles.
(7) Acid coagulation/isoelectric precipitation: The light phase from the centrifuge or filtrate from the filter is pumped into another tank where acid is added to bring the pH down to the isoelectric point of the proteins.
(8) Protein separation: The acidified suspension is subjected to another separation step where the coagulated/precipitated protein is separated from other components.
(9) Protein washing: Water is added to the precipitated protein at the same isoelectric pH and the mixture stirred for a few minutes. This washes the protein and removes non-protein material that may have coprecipitated and/or separated with it. More washing results in higher protein content of the final product, but there is an inverse relationship between the number or extent of washings and the yield of protein.
(10) Protein separation and dewatering: The precipitated and washed protein is separated and partially dewatered, using filters or centrifuges.
(11) Neutralization: Water and alkali (e.g., NaOH) is added to the precipitated protein to bring the protein up to approximately pH 7. [Note: Steps 10 and 11 could be done in either order].
(12) Dehydration: The protein solution is dried, usually by spray drying.
(13) Fiber-starch separation: From step (6), the heavy phase containing the carbohydrate macromolecules (fiber and starch) go through multiple separation processes to separate the fiber (using screens of the appropriate mesh size) followed by centrifuges (if needed to concentrate the crude starch stream) and hydrocyclones to wash the starch and purify it. The starch stream is dewatered and dried. [In some cases, e.g., corn wet-milling, the fiber is separated from the degermed corn meal immediately after the degermination operation. The de-fibered stream containing primarily starch and protein is then sent to a centrifugal protein-starch separator].
These conventional methods are time-consuming, they sometimes result in products with poor functional properties, and can generate a whey-like waste stream which contains some of the proteins and nutraceuticals. An increasing concern these days is that large quantities of water are required, especially to wash the curd (the precipitated protein) to remove as much of the adhering non-protein components. Since most concentrates and isolates are dried, every kg of water added is a kg of water that must be removed, usually by energy-intensive dehydration techniques. The waste water in a soy isolate plant has a high BOD (2000-10,000 ppm) and low solids level (less than 0.5%, usually). Discharging the waste water usually results in heavy penalties. Recycling the waste water within the plant will usually require it to be cleaned up to prevent carryover of off-flavors and undesirable components, which will build up in the product.
Membrane technology provides an opportunity to overcome many of these limitations. e.g., see soy protein concentrates and isolates by membrane technology.